Understanding Catalysts for Green Fuels: How Oxygen-Containing Molecules Interact with MoS₂
PhD student Martin Hedevang from iMAT and Aarhus University has used advanced surface science tools to learn how MoS₂-based catalysts behave under hydrodeoxygenation conditions. His research brings new insight into how these catalysts degrade in realistic environments, supporting efforts to develop greener fuels for aviation and shipping.
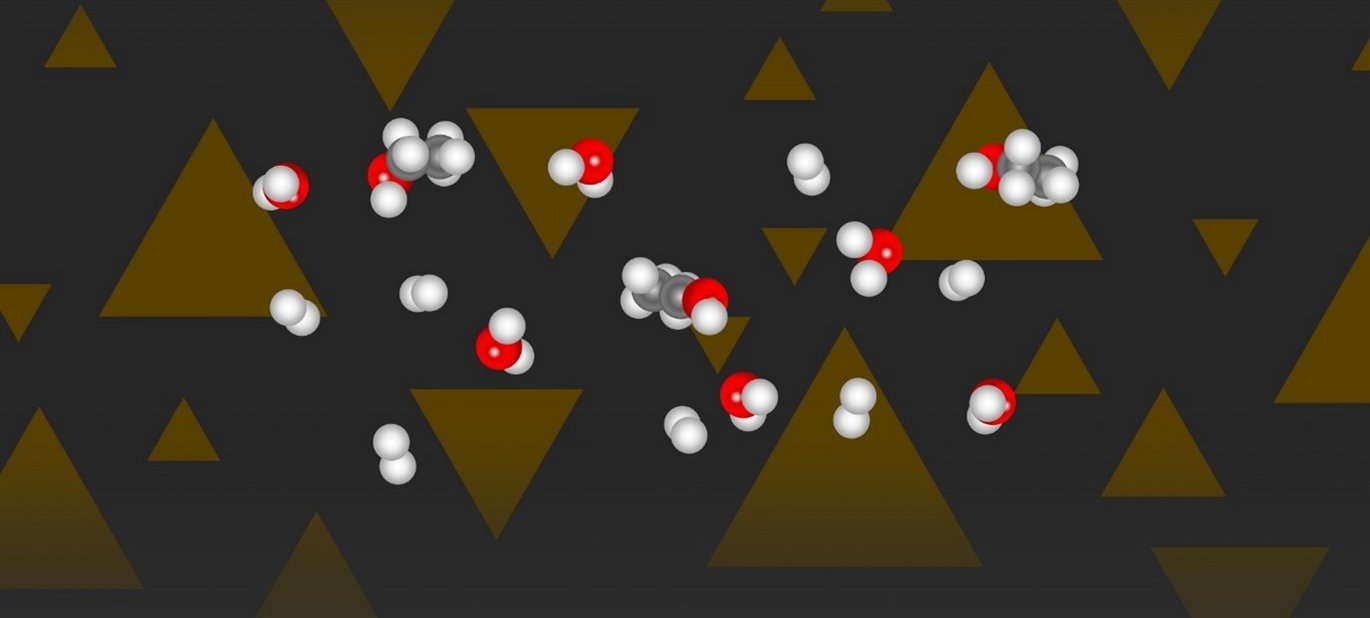
The aviation and shipping sectors remain some of the hardest to decarbonize. While road transport is transitioning toward electrification, long-distance travel requires liquid fuels, ideally produced from sustainable sources like biomass, as these have a high gravimetric energy density. Pyrolysis of biomass yields a liquid known as bio-oil. However, this oil must be upgraded through hydrodeoxygenation before it can be used in engines. This process depends on a catalyst that can withstand complex and harsh chemical environments, something current materials still struggle with.
Understanding What Happens on the Surface of Catalysts
In his PhD project, Martin focused on MoS₂-based catalysts, widely used in hydrodeoxygenation. He explored how the catalysts behave in the presence of water, ethanol, and other simple oxygen-containing molecules. His results show that the morphology and chemical composition of the catalyst is sensitive to the gas composition. Furthermore, he worked on models describing mechanisms present during hydrodeoxygenation.
The research is based on a series of in-depth surface science studies conducted using model catalyst systems under near-operational conditions. These studies investigate:
- Substrate-dependent reduction of MoS₂ in hydrogen
- The effect of water exposure on catalyst structure and phase
- Interaction of alcohols with MoS₂ under near-ambient pressures
To isolate the effects of individual functional groups, he used simple model compounds like ethanol and ethyl formate, mimicking components commonly found in bio-oils. This approach made it possible to directly observe how different oxygen moieties affect catalyst degradation pathways.
How Did They Do It?
To investigate how catalysts behave in chemically reactive environments, Martin combined a set of surface science techniques that allowed them to observe the catalyst’s evolution in real time and at the atomic scale.
- X-ray photoelectron spectroscopy (XPS) was used throughout the work to follow changes in the chemical state of MoS₂. XPS helped identify how sulfur was lost or exchanged, and how oxidation states evolved during reduction and reaction.
- Scanning tunneling microscopy (STM) made it possible to visualize the shape and structure of individual nanoparticles on flat model surfaces. This technique was especially useful for tracking how particle edges and basal planes responded to exposure, for example revealing etching effects caused by water or adsorption patterns of ethanol.
- Near-ambient pressure (NAP) environments were used to simulate realistic reaction conditions, a step beyond the ultra-high vacuum settings normally used in surface science. By performing STM and XPS at higher pressures, it was possible to observe how catalysts behave in environments that more closely mimic industrial HDO processing.
These methods together enabled the tracking of both the chemical and structural evolution of MoS₂ in the presence of specific molecules.
Key Findings: Investigating Reactions at the Atomic Scale
Using surface science techniques, the study explores how MoS₂ nanoparticles respond when exposed to hydrogen, water, and oxygen-containing molecules such as ethanol and ethyl formate. The research shows that each of these molecules interacts differently with the catalyst:
- Water tends to react at the edges of the nanoparticles, causing an oxygen/sulfur exchange and resulting in the formation of a distinct oxide phase.
- Ethanol and ethyl formate not only reduce the MoS₂ but also affect the basal plane, a part of the surface typically thought to be chemically inert.
These findings underscore the importance of studying how different oxygen-bearing functional groups affect catalyst behavior, as they appear to impact the structure and stability of the catalyst in different ways.
Why Does It Matter?
Understanding how real-world molecules interact with catalyst surfaces is essential for improving the durability and selectivity of materials used in green fuel production. While current research often focuses on optimizing performance, this study provides a look at how deactivation begins at the atomic level.
By identifying how different oxygen moieties influence the active sites of MoS₂, this work supports efforts to design more resilient catalysts for the HDO process, helping to make bio-based fuels more viable for large-scale use in aviation and shipping.
What’s Next? Stay Tuned!
The results of Martin’s PhD are currently in preparation for publication. Stay tuned for more as the findings are released later this year!
If you are working on related materials or applications, we invite you to reach out to our Center Manager to discuss potential collaborations or shared research opportunities.