Stabilizing Cobalt for More Efficient Water Splitting
The future of clean energy depends on developing highly efficient catalysts for water splitting. A new study from Aarhus University including several iMAT members explores how cobalt atoms can be stabilized within a carbon nitride framework, improving their performance in the oxygen evolution reaction. By uncovering key atomic-scale interactions, this research opens the door to more durable and cost-effective catalyst materials.
Hydrogen is emerging as a key player in the transition to clean energy, but efficient and scalable production remains a challenge. A recent study published in Physical Chemistry Chemical Physics sheds light on how stabilizing cobalt atoms within a monolayer carbon nitride framework can significantly enhance the oxygen evolution reaction (OER), a crucial step in water splitting for hydrogen generation. Using X-ray spectroscopy, the researchers have mapped the atomic interactions that govern the efficiency and durability of these catalysts, providing new insights into catalyst design for sustainable energy applications.
How Cobalt Finds Its Place in the Catalyst
In the quest for efficient hydrogen production, the OER represents a major bottleneck due to its sluggish reaction kinetics. To accelerate this process, catalysts are required to facilitate the breakdown of water molecules into oxygen and hydrogen. In this study, researchers investigated how cobalt atoms can be stabilized within a monolayer carbon nitride framework called CoCN. They have studied the effects of stabilizing cobalt on both catalytic efficiency and long-term durability.
Through X-ray spectroscopy, the team analyzed the atomic-level interactions between cobalt and the surrounding carbon nitride matrix during the OER. The researchers could show that oxidized cobalt nanoparticles on the surface of the catalyst are reorganizing themselves as CoOOH and anchoring in the pockets of carbon nitride framework (Figure 1).
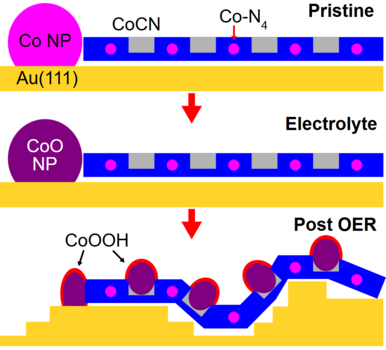
How Did They Do It?
To understand how cobalt stabilizes within a monolayer carbon nitride framework, the researchers combined precise material synthesis with advanced X-ray spectroscopy techniques to analyze the atomic structure and electronic properties of the catalyst.
- X-ray Spectroscopy Analysis: Using X-ray absorption spectroscopy (XAS) and X-ray photoelectron spectroscopy (XPS), they investigated the chemical state and coordination environment of cobalt at an atomic level. This allowed them to determine how the metal atoms interact with the surrounding carbon nitride structure.
- Tracking the Catalyst’s Behavior: By examining how the oxidation state and electronic structure of cobalt evolved under reaction conditions, they identified key factors contributing to its stability and catalytic efficiency.
- Comparing Performance: The results were analyzed to compare the activity and durability of the cobalt-doped carbon nitride with other known catalysts, confirming its enhanced performance for the OER.
This systematic approach provided a detailed atomic-scale understanding of how cobalt functions within the carbon nitride framework.
Unraveling the Role of Cobalt in Water Splitting
Their findings reveal that a carefully tuned environment improves the catalytic activity of the material, making it more efficient and stable during the OER process. The study demonstrates that cobalt atoms integrate into a monolayer carbon nitride, preventing agglomeration and ensuring long-term usage. The presence of nitrogen creates a bonding environment for cobalt, preventing the cobalt from agglomerating. This leads to an improved performance of the catalyst. In contrast, when cobalt is alone, without carbon nitride, it oxidizes and agglomerates resulting in decreased efficiency as shown in Figure 2.
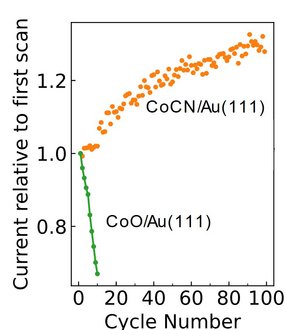
A Step Toward Scalable Green Hydrogen
Hydrogen is widely regarded as a clean energy carrier that can support the decarbonization of multiple industries, from transportation to chemical production. By addressing the challenges of catalyst stability and efficiency, this study marks an important step toward developing cost-effective catalysts for hydrogen production. The insights gained from this research could lead to new generations of catalysts tailored for sustainable energy applications, contributing to a cleaner and more efficient energy future.
Interested?
You can explore the full study in Physical Chemistry Chemical Physics . Otherwise, feel free to explore the other research topics we work on at iMAT.
If you are working on related materials or applications, we invite you to reach out to our Center Manager to discuss potential collaborations or shared research opportunities.
Anders K. Vestergaard , Jens Jakob Gammelgaard , Zhaozong Sun , Siqi Zhao , Zheshen Li , Nina Lock , Kim Daasbjerg and Jeppe Vang Lauritsen